Recent Research Highlights
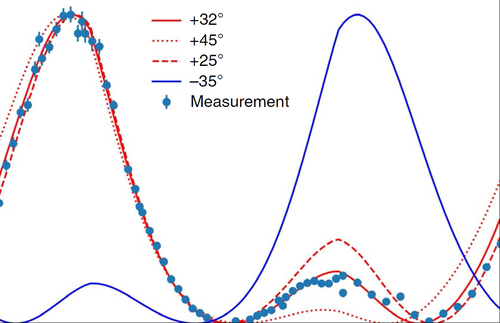
The magnetic behaviour of alpha-RuCl3 is known to be very unusual, with excitations similar to those expected in the Kitaev quantum spin liquid – a much sought-after phase of matter that would have potential applications to quantum computing. (See below.) Despite explosive interest in this material, the magnetic Hamiltonian appropriate to describe α-RuCl3 has not been agreed upon.
In our recent Resonant Elastic X-ray Scattering (REXS) investigation, we found that ferromagnetic Kitaev and antiferromagnetic Gamma (off-diagonal symmetric exchange) terms are two dominant energy scales essential for describing the magnetism of α-RuCl3. Furthermore, the large Gamma interaction in addition to the Kitaev interaction, found in our study provides a natural solution for the long-standing mystery regarding the large magnetic anisotropy observed in this material.
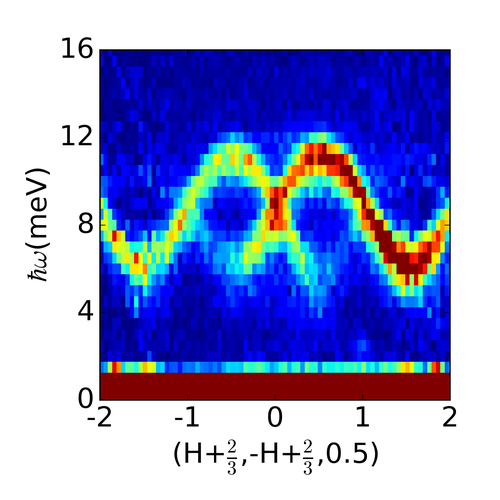
Graphene has fascinated condensed-matter physicists for decades because of its intriguing electronic properties. Unlike an ordinary metal, where the energy of an electron increases quadratically with momentum, electrons in graphene have a linear dispersion relation very much like photons, implying that they move through the lattice as if they are massless. Interactions between these unusual electrons in graphene, sometimes called Dirac electrons, are key to explaining its many strange electronic properties.
Many types of particles other than electrons are known to exist in condensed-matter systems. For example, in an ordered magnet, a spin fluctuation can propagate coherently through the lattice just like a particle, known as a “magnon.”
In our recent study of a honeycomb lattice quantum magnet CoTiO3, we found that the energy-momentum (or dispersion) relation for magnons shows a striking resemblance to that of an electron in graphene. Our discovery makes CoTiO3 an ideal platform to study Dirac bosons.
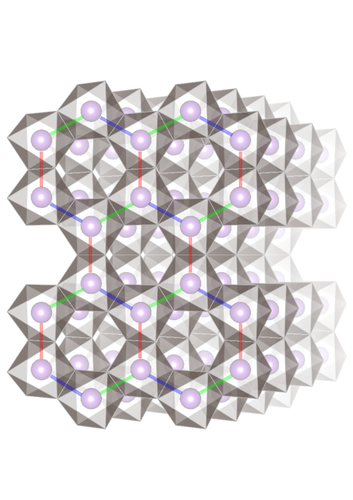
Kitaev model was first proposed as an exactly solvable model with many remarkable qualities that are of interest to quantum computation. In this model, the spin on a honeycomb lattice are coupled with Ising-like interaction that depends on the spatial bond direction. This model is also interesting to quantum magnetism, since its ground state is an exactly solvable quantum spin liquid. One can think of this as another example of frustrated magnetism. However, this purely theoretical model has made a significant inroad into experimental condensed matter physics when researchers realized that Kitaev physics can be found in materials with strong spin-orbit coupling. In particular, honeycomb lattice iridates such as Na2IrO3 have been drawing much attention as the most promising platform to study quantum magnetism associated with Kitaev spin liquid. In a recent series of papers, our group proposed that alpha-RuCl3 (structure shown) is another candidate material to study Kitaev physics. (See Plumb et al. PRB 2014). Many researchers around the world are now investigating this material.
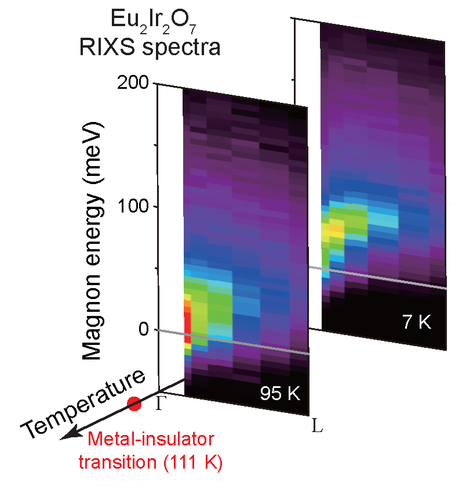
Iridium oxides (iridates) exhibit many diverse and interesting electronic phases due to interplays between electron correlations and spin-orbit coupling effects. One possible such topological phase is the Weyl semimetal state, which in pyrochlore iridates can exist in the all-in–all-out (AIAO) magnetic state. Because the possible presence of this phase in pyrochlore iridates depends on the strength of the electron correlation, which remains a matter of continuing debate, a research team sought to understand the degree of electron correlation in the pyrochlore iridate Eu2Ir2O7 as a crucial first step toward assessing the topological phase of this class of materials. In examining this issue, the researchers studied the temperature-dependent evolution of the magnetic excitation spectrum in a highly insulating single crystal of Eu2Ir2O7 utilizing resonant inelastic x-ray scattering (RIXS) at the APS.
RIXS is a particularly apt way to probe the properties of correlated electrons. RIXS is sensitive to collective spin excitations called “magnons,” which form a propagating wave of spinning magnetic moments. Magnons carry information about the strength of the coupling between neighboring electron spins, which in turn sheds light on the degree of electron correlation. Magnons in complex antiferromagnets like Eu2Ir2O7 cannot be probed with other x-ray spectroscopic techniques.
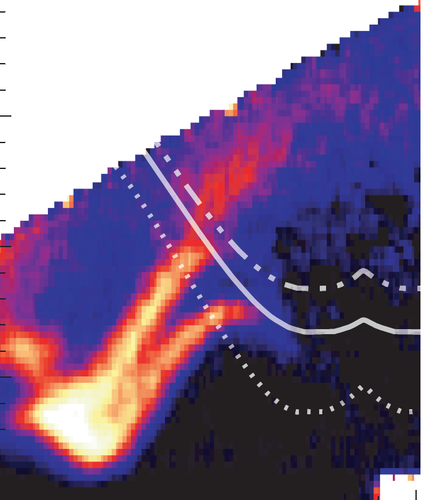
Physicists often describe a solid as a zoo in which many different species of so-called quasiparticles are roaming. These “virtual” particles are not real elementary particles, but they do act like one, and quasiparticles do have well-defined mass and velocity. For example, heat and sound are both transmitted by quasiparticles called phonons through our living room window. In our study, we studied a system of magnetic quasiparticles called triplons, which is attractive to physicists since they usually exist only at temperatures close to absolute zero. At such a low temperature, not many other species of quasiparticles are present, and therefore fundamental properties of quasiparticles can be studied without being bothered by other species. We reported that the interaction between these magnetic quasiparticles is unusually strong in the particular quantum magnet we studied. This turns out to be an important ingredient that allows three distinct subspecies of triplons to behave very differently. One of these decays slowly, while the second one disappears immediately. However, the third one actually tries to avoid entering the decay region altogether by altering its path and speed. The latter behaviour is quite reminiscent of a quantum mechanical phenomenon called avoided level crossing often found in atomic systems, but in this case the repulsion occurs between a quasiparticle and a region of phase space. Our study allows one to learn about how strongly interacting quasiparticles decay, and can be of general importance for understanding particle decay in other areas of physics.